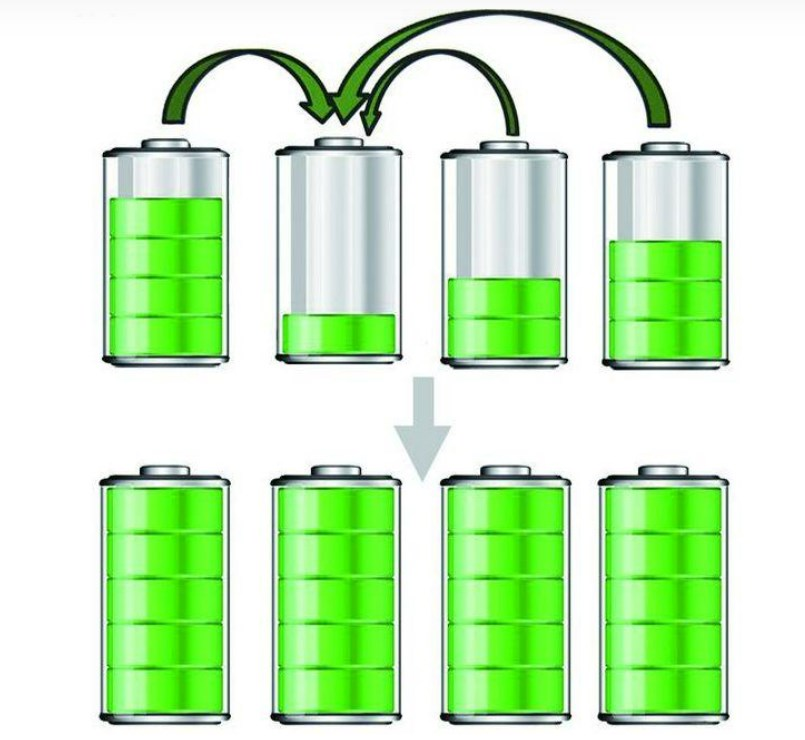
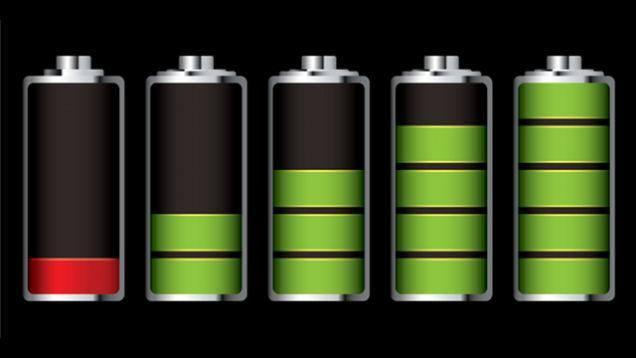
The concept of cell balancing is probably familiar to most of us. This is mainly because the current consistency of cells is not good enough, and balancing helps improve this. Just like you can't find two identical leaves in the world, you also can't find two identical cells. So, ultimately, balancing is to address the shortcomings of cells, serving as a compensatory measure.
What Aspects Show Cell Inconsistency?
There are four main aspects: SOC (State of Charge), internal resistance, self-discharge current, and capacity. However, balancing can't completely solve these four discrepancies. Balancing can only compensate for SOC differences, incidentally addressing self-discharge inconsistencies. But for internal resistance and capacity, balancing is powerless.
How Is Cell Inconsistency Caused?
There are two main reasons: one is the inconsistency caused by cell production and processing, and the other is the inconsistency caused by the cell usage environment. Production inconsistencies arise from factors like processing techniques and materials, which is a simplification of a very complex issue. Environmental inconsistency is easier to understand, as each cell's position in the PACK is different, leading to environmental differences such as slight variations in temperature. Over time, these differences accumulate, causing cell inconsistency.
How Does Balancing Work?
As mentioned earlier, balancing is used to eliminate SOC differences among cells. Ideally, it keeps each cell's SOC the same, allowing all cells to reach the upper and lower voltage limits of charge and discharge simultaneously, thus increasing the usable capacity of the battery pack. There are two scenarios for SOC differences: one is when cell capacities are the same but SOCs are different; the other is when cell capacities and SOCs are both different.
The first scenario (leftmost in the illustration below) shows cells with the same capacity but different SOCs. The cell with the smallest SOC reaches the discharge limit first (assuming 25% SOC as the lower limit), while the cell with the largest SOC reaches the charge limit first. With balancing, all cells maintain the same SOC during charge and discharge.
The second scenario (second from the left in the illustration below) involves cells with different capacities and SOCs. Here, the cell with the smallest capacity charges and discharges first. With balancing, all cells maintain the same SOC during charge and discharge.
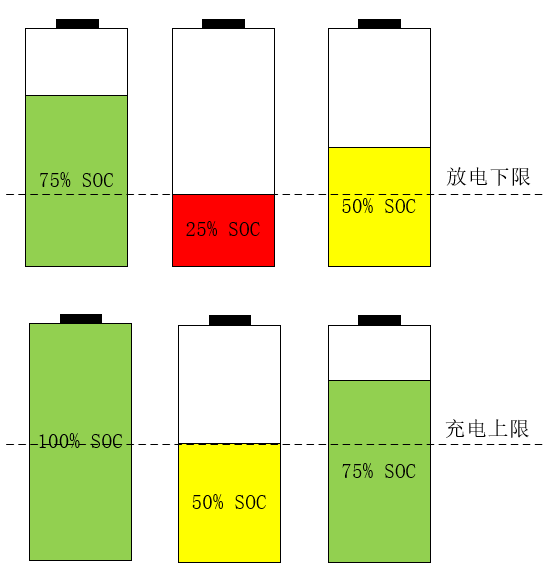
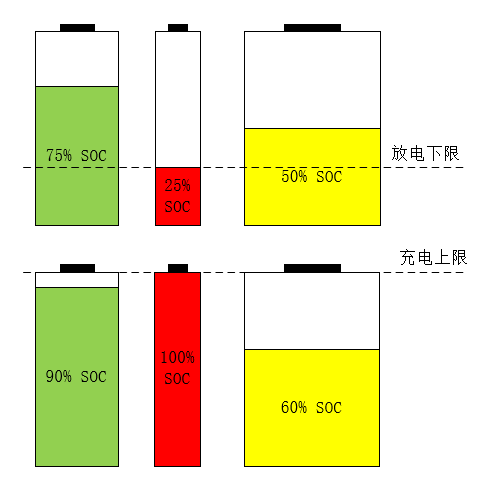
The Importance of Balancing
Balancing is a crucial function for current cells. There are two types of balancing: active balancing and passive balancing. Passive balancing uses resistors for discharge, while active balancing involves the flow of charge between cells. There is some debate about these terms, but we won't go into that. Passive balancing is more commonly used in practice, while active balancing is less common.
Deciding the Balancing Current for BMS
For passive balancing, how should the balancing current be determined? Ideally, it should be as large as possible, but factors like cost, heat dissipation, and space require a compromise.
Before choosing the balancing current, it's important to understand whether the SOC difference is due to scenario one or scenario two. In many cases, it's closer to scenario one: cells start with almost identical capacity and SOC, but as they are used, especially due to differences in self-discharge, each cell's SOC gradually becomes different. Therefore, the balancing capability should at least eliminate the impact of self-discharge differences.
If all cells had identical self-discharge, balancing wouldn't be necessary. But if there's a difference in self-discharge current, SOC differences will arise, and balancing is needed to compensate for this. Additionally, since the average daily balancing time is limited while self-discharge continues daily, the time factor must also be considered.
Post time: Jul-05-2024